Whole Food Plant Based Diet Criticism
Abstract
Western societies notice an increasing interest in plant-based eating patterns such as vegetarian and vegan, yet potential effects on the body and brain are a matter of debate. Therefore, we systematically reviewed existing human interventional studies on putative effects of a plant-based diet on the metabolism and cognition, and what is known about the underlying mechanisms. Using the search terms "plant-based OR vegan OR vegetarian AND diet AND intervention" in PubMed filtered for clinical trials in humans retrieved 205 studies out of which 27, plus an additional search extending the selection to another five studies, were eligible for inclusion based on three independent ratings. We found robust evidence for short- to moderate-term beneficial effects of plant-based diets versus conventional diets (duration ≤ 24 months) on weight status, energy metabolism and systemic inflammation in healthy participants, obese and type-2 diabetes patients. Initial experimental studies proposed novel microbiome-related pathways, by which plant-based diets modulate the gut microbiome towards a favorable diversity of bacteria species, yet a functional "bottom up" signaling of plant-based diet-induced microbial changes remains highly speculative. In addition, little is known, based on interventional studies about cognitive effects linked to plant-based diets. Thus, a causal impact of plant-based diets on cognitive functions, mental and neurological health and respective underlying mechanisms has yet to be demonstrated. In sum, the increasing interest for plant-based diets raises the opportunity for developing novel preventive and therapeutic strategies against obesity, eating disorders and related comorbidities. Still, putative effects of plant-based diets on brain health and cognitive functions as well as the underlying mechanisms remain largely unexplored and new studies need to address these questions.
Introduction
Background
Western societies notice an increasing interest in plant-based eating patterns such as avoiding meat or fish or fully excluding animal products (vegetarian or vegan, see Fig. 1). In 2015, around 0.4−3.4% US adults, 1−2% British adults, and 5−10% of German adults were reported to eat largely plant-based diets1,2,3,4, due to various reasons (reviewed in ref. 5). Likewise, the number of scientific publications on PubMed (Fig. 2) and the public popularity as depicted by Google Trends (Fig. 3) underscore the increased interest in plant-based diets. This increasing awareness calls for a better scientific understanding of how plant-based diets affect human health, in particular with regard to potentially relevant effects on mental health and cognitive functions.
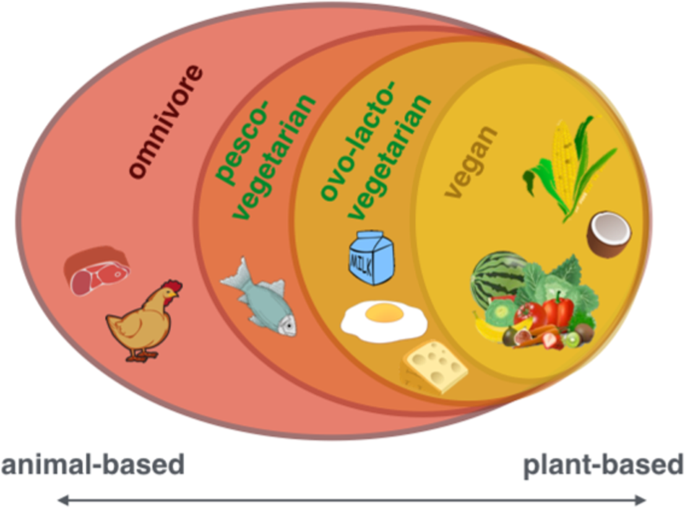
From left to right: including all food items (omnivore), including all except for meat (pesco-vegetarian) or meat and fish (ovo-lacto-vegetarian) to including only plant-based items (vegan)
Full size image
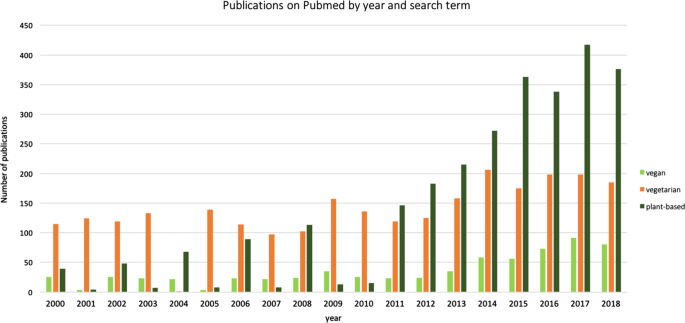
Frequency of publications on PubMed including the search terms "vegan" (in light green), vegetarian (in orange) and plant-based (dark green)—accessed on 19 April 2019
Full size image
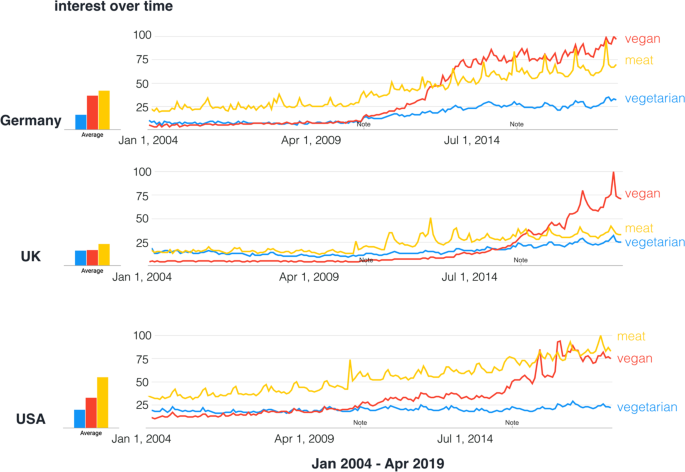
Note indicates technical improvements implemented by Google Trends. Data source: Google Trends. Search performed on 18 April 2019
Full size image
Study aims
A potential effect of plant-based diets on mortality rate remains controversial: large epidemiological studies like the Adventist studies (n = 22,000−96,000) show a link between plant-based diets, lower all-cause mortality and cardiovascular diseases6,7, while other studies like the EPIC-Oxford study and the "45 and Up Study" (n = 64,000−267,000) show none8,9. Yet, many, but not all, epidemiological and interventional human studies in the last decades have suggested that plant-based diets exert beneficial health effects with regard to obesity-related metabolic dysfunction, type 2 diabetes mellitus (T2DM) and chronic low-grade inflammation (e.g. refs. 6,7,10,11, for reviews, see refs. 12,13,14,15,16,17,18). However, while a putative link between such metabolic alterations and brain health through pathways which might include diet-related neurotransmitter precursors, inflammatory pathways and the gut microbiome19 becomes increasingly recognized, the notion that plant-based diets exert influence on mental health and cognitive functions appears less documented and controversial20,21,22,23,24. We therefore systematically reviewed the current evidence based on available controlled interventional trials, regarded as the gold standard to assess causality, on potential effects of plant-based diets on (a) metabolic factors including the microbiome and (b) neurological or psychiatric health and brain functions. In addition, we aimed to evaluate potential underlying mechanisms and related implications for cognition.
Methods
We performed a systematic PubMed search with the following search terms "plant-based OR vegan OR vegetarian AND diet AND intervention" with the filter "clinical trial" and "humans", preregistered at PROSPERO (CRD42018111856; https://www.crd.york.ac.uk/PROSPERO/display_record.php?RecordID=111856) (Suppl. Fig. 1). PubMed was used as search engine because it was esteemed to yield the majority of relevant human clinical trials from a medical perspective. Exclusion criteria were insufficient design quality (such as lack of a control group), interventions without a plant-based or vegetarian or vegan diet condition, intervention with multiple factors (such as exercise and diet), and the exclusive report of main outcomes of no interest, such as dietary compliance, nutrient intake (such as vitamins or fiber intake), or nonmetabolic (i.e., not concerning glucose metabolism, lipid profile, gastrointestinal hormones or inflammatory markers) or non-neurological/psychiatric disease outcomes (e.g. cancer, caries).
Studies were independently rated for eligibility into the systematic review by three authors based on reading the abstract and, if needed, methods or other parts of the publication. If opinions differed, a consensus was reached through discussion of the individual study. This yielded 27 eligible out of 205 publications; see Table 1 for details. To increase the search radius for studies dealing with microbial and neurological/psychiatric outcomes, we deleted the search term "intervention", which increased the number of studies by around one third, and checked for studies with "microbiome/microbiota", "mental", "cognitive/cognition" or "psychological/psychology" in the resulting records. Through this, we retrieved another five studies included in Table 1. Further related studies were reviewed based on additional nonsystematic literature search.
Full size table
Section I: Effects of plant-based diets on body and brain outcomes
Results based on interventional studies on metabolism, microbiota and brain function
Overall, the vast majority of studies included in this systematic review reported a short-term beneficial effect of plant-based dietary interventions (study duration 3−24 months) on weight status, glucose, insulin and/or plasma lipids and inflammatory markers, whereas studies investigating whether plant-based diets affect microbial or neurological/psychiatric disease status and other brain functions were scarce and rather inconclusive (Table 1).
More specifically, 19 out of 32 studies dealing with T2DM and/or obese subjects and seven out of 32 dealing with healthy subjects observed a more pronounced weight loss and metabolic improvements, such as lowering of glycated hemoglobin (HbA1c)—a long-term marker for glucose levels—decreased serum levels of low-density (LDL) and high-density lipoproteins (HDL) and total cholesterol (TC), after a plant-based diet compared to an omnivore diet. This is largely in line with recent meta-analyses indicating beneficial metabolic changes after a plant-based diet25,26,27.
For example, Lee et al. found a significantly larger reduction of HbA1c and lower waist circumference after vegan compared to conventional dieting28. Jenkins et al. found a disease-attenuating effect in hyperlipidemic patients after 6 months adopting a low-carbohydrate plant-based diet compared to a high-carbohydrate lacto-ovo-vegetarian diet29,30. However, lower energy intake in the vegan dieters might have contributed to these effects. Yet, while a plant-based diet per se might lead to lower caloric intake, other studies observed nonsignificant trends toward higher effect sizes on metabolic parameters after a vegan diet, even when caloric intake was comparable: two studies in T2DM patients31,32 compared calorie-unrestricted vegan or vegetarian to calorie-restricted conventional diets over periods of 6 months and 1.5 years, respectively, in moderate sample sizes (n ~ 75−99) with similar caloric intake achieved in both diet groups. Both studies indicated stronger effects of plant-based diets on disease status, such as reduced medication, improved weight status and increased glucose/insulin sensitivity, proposing a diabetes-preventive potential of plant-based diets. Further, a five-arm study comparing four types of plant-based diets (vegan, vegetarian, pesco-vegetarian, semi-vegetarian) to an omnivore diet (total n = 63) in obese participants found the most pronounced effect on weight loss for a vegan diet (−7.5 ± 4.5% of total body weight)33. Here, inflammation markers conceptualized as the dietary inflammatory index were also found to be lower in vegan, vegetarian and pesco-vegetarian compared to semi-vegetarian overweight to obese dieters33.
Intriguingly, these results28,29,30,31,32,33 cohesively suggest that although caloric intake was similar across groups, participants who had followed a vegan diet showed higher weight loss and improved metabolic status.
As a limitation, all of the reviewed intervention studies were carried out in moderate sample sizes and over a period of less than 2 years, disregarding that long-term success of dietary interventions stabilizes after 2−5 years only34. Future studies with larger sample sizes and tight control of dietary intake need to confirm these results.
Through our systematic review we retrieved only one study that added the gut microbiome as novel outcome for clinical trials investigating the effects of animal-based diets compared to plant-based diets. While the sample size was relatively low (n = 10, cross-over within subject design), it showed that changing animal- to plant based diet changed gut microbial activity towards a trade-off between carbohydrate and protein fermentation processes within only 5 days35. This is in line with another controlled-feeding study where microbial composition changes already occurred 24 h after changing diet (not exclusively plant-based)36. However, future studies incorporating larger sample sizes and a uniform analysis approach of microbial features need to further confirm the hypothesis that a plant-based diet ameliorates microbial diversity and health-related bacteria species.
Considering neurological or psychiatric diseases and brain functions, the systematic review yielded in six clinical trials of diverse clinical groups, i.e. migraine, multiple sclerosis, fibromyalgia and rheumatoid arthritis. Here, mild to moderate improvement, e.g. measured by antibody levels, symptom improvement or pain frequency, was reported in five out of six studies, sometimes accompanied by weight loss37,38,39,40 (Table 1). However, given the pilot character of these studies, indicated by small sample sizes (n = 32−66), lack of randomization37, or that the plant-based diet was additionally free of gluten40, the evidence is largely anecdotal. One study in moderately obese women showed no effects on psychological outcomes41, two studies with obese and nonobese healthy adults indicated improvements in anxiety, stress and depressive symptom scores23,24. Taken together, the current evidence based on interventional trials regarding improvements of cognitive and emotional markers and in disease treatment for central nervous system disorders such as multiple sclerosis or fibromyalgia remains considerably fragmentary for plant-based diets.
Among observational studies, a recent large cross-sectional study showed a higher occurrence of depressive symptoms for vegetarian dieters compared to nonvegetarians20. Conversely, another observational study with a sample of about 80% women found a beneficial association between a vegan diet and mood disturbance24.
Overall, the relationship between mental health (i.e. depression) and restrictive eating patterns has been the focus of recent research20,21,22,24,42; however, causal relationships remain uninvestigated due to the observational design.
Underlying mechanisms linking macronutrient intake to metabolic processes
On the one hand, nutrient sources as well as their intake ratios considerably differ between plant-based and omnivore diets (Suppl. Table 1), and on the other hand, dietary micro- and macromolecules as well as their metabolic substrates affect a diversity of physiological functions, pointing to complex interdependencies. Thus, it seems difficult to nail down the proposed beneficial effects of a plant-based diet on metabolic status to one specific component or characteristic, and it seems unlikely that the usually low amount of calories in plant-based diets could explain all observed effects. Rather, plant-based diets might act through multiple pathways, including better glycemic control43, lower inflammatory activity44 and altered neurotransmitter metabolism via dietary intake45 or intestinal activity46 (Fig. 4).
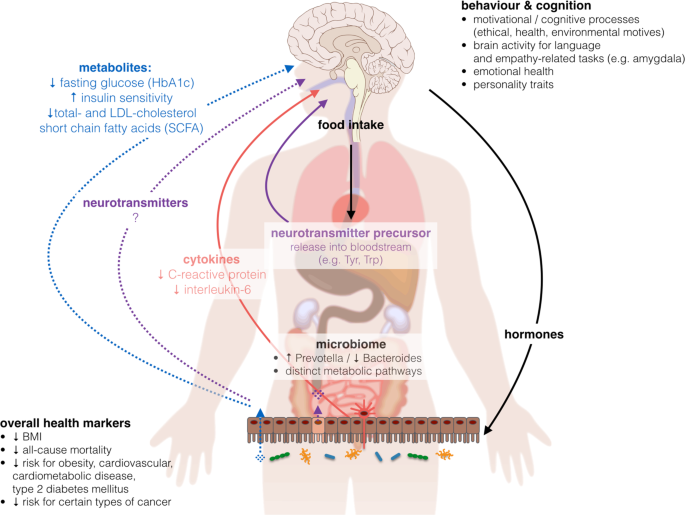
BMI body-mass-index, HbA1c hemoglobin A1c, LDL-cholesterol low-density lipoprotein cholesterol, Trp tryptophan, Tyr tyrosine. Images from commons.wikimedia.org, "Brain human sagittal section" by Lynch 2006 and "Complete GI tract" by Häggström 2008, "Anatomy Figure Vector Clipart" by http://moziru.com
Full size image
On the macronutrient level, plant-based diets feature different types of fatty acids (mono- and poly-unsaturated versus saturated and trans) and sugars (complex and unrefined versus simple and refined), which might both be important players for mediating beneficial health effects18. On the micronutrient level, the EPIC-Oxford study provided the largest sample of vegan dieters worldwide (n(vegan) = 2396, n(total) = 65,429) and showed on the one hand lower intake of saturated fatty acids (SFA), retinol, vitamin B12 and D, calcium, zinc and protein, and on the other hand higher intake of fiber, magnesium, iron, folic acid, vitamin B1, C and E in vegan compared to omnivore dieters47. Other studies confirmed the variance of nutrient intake across dietary groups, i.e. omnivores, vegetarians and vegans, showing the occurrence of critical nutrients for each group48,49. Not only the amount of SFA but also its source and profile might be important factors regulating metabolic control (reviewed in ref. 14), for example through contributing to systemic hyperlipidemia and subsequent cardiovascular risk. Recently, it has been shown in a 4-week intervention trial that short-term dietary changes favoring a diet high in animal-based protein may lead to an increased risk for cardiovascular derangements mediated by higher levels of trimethylamine N-oxide (TMAO), which is a metabolite of gut bacteria-driven metabolic pathways50.
Secondly, high fiber intake from legumes, grains, vegetables and fruits is a prominent feature of plant-based diets (Table 1), which could induce beneficial metabolic processes like upregulated carbohydrate fermentation and downregulated protein fermentation35, improved gut hormonal-driven appetite regulation51,52,53,54,55, and might prevent chronic diseases such as obesity and T2DM by slowing down digestion and improving lipid control56. A comprehensive review including evidence from 185 prospective studies and 58 clinical trials concluded that risk reduction for a myriad of diseases (incl. CVD, T2DM, stroke incidence) was greatest for daily fiber intake between 25 and 29 g57. Precise evidence for underlying mechanisms is missing; however, more recently it has been suggested that high fiber intake induces changes on the microbial level leading to lower long-term weight gain58, a mechanism discussed below.
The reason for lower systemic inflammation in plant-based dieters could be due to the abundance of antiinflammatory molecule intake and/or avoidance of proinflammatory animal-derived molecules. Assessing systemic inflammation is particularly relevant for medical conditions such as obesity, where it has been proposed to increase the risk for cardiovascular disease59,60. In addition, higher C-reactive protein (CRP) and interleukin-6 (IL-6) levels have been linked with measures of brain microstructure, such as microstructural integrity and white matter lesions61,62,63 and higher risk of dementia64, and recent studies point out that a diet-related low inflammatory index might also directly affect healthy brain ageing65,66.
Interventional studies that focus on plant- versus meat-based proteins or micronutrients and potential effects on the body and brain are lacking. A meta-analysis including seven RCTs and one cross-sectional studies on physical performance and dietary habits concluded that a vegetarian diet did not adversely influence physical performance compared to an omnivore diet67. An epidemiological study by Song et al.11 estimated that statistically replacing 3% of animal protein, especially from red meat or eggs, with plant protein would significantly improve mortality rates. This beneficial effect might however not be explained by the protein source itself, but possibly by detrimental components found in meat (e.g. heme-iron or nitrosamines, antibiotics, see below).
Some studies further hypothesized that health benefits observed in a plant-based diet stem from higher levels of fruits and vegetables providing phytochemicals or vitamin C that might boost immune function and eventually prevent certain types of cancer68,69,70. A meta-analysis on the effect of phytochemical intake concluded a beneficial effect on CVD, cancer, overweight, body composition, glucose tolerance, digestion and mental health71. Looking further on the impact of micronutrients and single dietary compounds, there is room for speculation that molecules, that are commonly avoided in plant-based diets, might affect metabolic status and overall health, such as opioid-peptides derived from casein72, pre- and probiotics73,74, carry-over antibiotics found in animal products75,76 or food-related carcinogenic toxins, such as dioxin found in eggs or nitrosamines found in red and processed meat77,78. Although conclusive evidence is missing, these findings propose indirect beneficial effects on health deriving from plant-based compared to animal-based foods, with a potential role for nonprotein substances in mediating those effects18. While data regarding chemical contaminant levels (such as crop pesticides, herbicides or heavy metals) in different food items are fragmentary only, certain potentially harmful compounds may be more (or less) frequently consumed in plant-based diets compared to more animal-based diets79. Whether these differences lead to systematic health effects need to be explored.
Taken together, the reviewed studies indicating effects of plant-based diets through macro- and micronutrient intake reveal both the potential of single ingredients or food groups (low SFA, high fiber) and the immense complexity of diet-related mechanisms for metabolic health. As proposed by several authors, benefits on health related to diet can probably not be viewed in isolation for the intake (or nonintake) of specific foods, but rather by additive or even synergistic effects between them (reviewed in refs. 12,80). Even if it remains a challenging task to design long-term RCTs that control macro- and micronutrient levels across dietary intervention groups, technological advancements such as more fine-tuned diagnostic measurements and automated self-monitoring tools, e.g. automatic food recognition systems81 and urine-related measures of dietary intake82, could help to push the field forward.
Nutrients of particular interest in plant-based diets
As described above, plant-based diets have been shown to convey nutritional benefits48,49, in particular increased fiber, beta carotene, vitamin K and C, folate, magnesium, and potassium intake and an improved dietary health index83. However, a major criticism of plant-based diets is the risk of nutrient deficiencies for specific micronutrients, especially vitamin B12, a mainly animal-derived nutrient, which is missing entirely in vegan diets unless supplemented or provided in B12-fortified products, and which seems detrimental for neurological and cognitive health when intake is low. In the EPIC-Oxford study about 50% of the vegan dieters showed serum levels indicating vitamin B12 deficiency84. Along other risk factors such as age85, diet, and plant-based diets in particular, seem to be the main risk factor for vitamin B12 deficiency (reviewed in ref. 86), and therefore supplementing vitamin B12 for these risk groups is highly recommended87. Vitamin B12 is a crucial component involved in early brain development, in maintaining normal central nervous system function88 and suggested to be neuroprotective, particularly for memory performance and hippocampal microstructure89. One hypothesis is that high levels of homocysteine, that is associated with vitamin B12 deficiency, might be harmful to the body. Vitamin B12 is the essential cofactor required for the conversion of homocysteine into nonharmful components and serves as a cofactor in different enzymatic reactions. A person suffering from vitamin B12 insufficiency accumulates homocysteine, lastly promoting the formation of plaques in arteries and thereby increasing atherothrombotic risk90, possibly facilitating symptoms in patients of Alzheimer's disease91. A meta-analysis found that vitamin B12 deficiency was associated with stroke, Alzheimer's disease, vascular dementia, Parkinson's disease and in even lower concentrations with cognitive impairment92, supporting the claim of its high potential for disease prevention when avoided or treated93. Further investigations and longitudinal studies are needed, possibly measuring holotranscobalamin (the active form of vitamin B12) as a more specific and sensitive marker for vitamin B12 status94, to examine in how far nonsupplementing vegan dieters could be at risk for cardiovascular and cognitive impairment.
Similar health dangers can stem from iron deficiency, another commonly assumed risk for plant-based dieters and other risk groups such as young women. A meta-analysis on 24 studies proposes that although serum ferritin levels were lower in vegetarians on average, it is recommended to sustain an optimal ferritin level (neither too low nor too high), calling for well-monitored supplementation strategies95. Iron deficiency is not only dependent on iron intake as such but also on complimentary dietary factors influencing its bioavailability (discussed in ref. 95). The picture remains complex: on the one hand iron deficiency may lead to detrimental health effects, such as impairments in early brain development and cognitive functions in adults and in children carried by iron-deficient mothers96 and a possible role for iron overload in the brain on cognitive impairment on the other hand97. One study showed that attention, memory and learning were impaired in iron-deficient compared to iron-sufficient women, which could be restored after a 4-month oral iron supplementation (n = 118)98. Iron deficiency-related impairments could be attributed to anemia as an underlying cause, possibly leading to fatigue, or an undersupply of blood to the brain or alterations in neurobiological and neuronal systems99 provoking impaired cognitive functioning.
This leads to the general recommendation to monitor health status by frequent blood tests, to consult a dietician to live healthily on a plant-based diet and to consider supplements to avoid nutrient deficiencies or nutrient-overdose-related toxicity. All in all, organizations such as the Academy of Nutrition and Dietetics100 and the German Nutrition Society do not judge iron as a major risk factor for plant-based dieters101.
Section II: Effects of diet on the gut microbiome
The link between diet and microbial diversity
Another putative mechanistic pathway of how plant-based diets can affect health may involve the gut microbiome which has increasingly received scientific and popular interest, lastly not only through initiatives such as the Human Microbiome Project102. A common measure for characterizing the gut community is enterotyping, which is a way to stratify individuals according to their gut bacterial diversity, by calculating the ratio between bacterial genera, such as Prevotella and Bacteroides103. While interventional controlled trials are still scarce, this ratio has been shown to be conclusive for differentiating plant-based from animal-based microbial profiles36. Specifically, in a sample of 98 individuals, Wu et al.36 found that a diet high in protein and animal fats was related to more Bacteroides, whereas a diet high in carbohydrates, representing a plant-based one, was associated with more Prevotella. Moreover, the authors showed that a change in diet to high-fat/low-fiber or to low-fat/high-fiber in ten individuals elicited a change in gut microbial enterotype with a time delay of 24 h only and remained stable over 10 days, however not being able to switch completely to another enterotype36. Another strictly controlled 30-day cross-over interventional study showed that a change in diet to either an exclusively animal-based or plant-based diet promoted gut microbiota diversity and genetic expression to change within 5 days35. Particularly, in response to adopting an animal-based diet, microbial diversity increased rapidly, even overshadowing individual microbial gene expression. Beyond large shifts in overall diet, already modest dietary modifications such as the daily consumption of 43 g of walnuts, were able to promote probiotic- and butyric acid-producing bacterial species in two RCTs, after 3 and 8 weeks respectively104,105, highlighting the high adaptability of the gut microbiome to dietary components. The Prevotella to Bacteroides ratio (P/B) has been shown to be involved in the success of dietary interventions targeting weight loss, with larger weight loss in high P/B compared to low P/B in a 6-month whole-grain diet compared to a conventional diet106. Only recently, other microbial communities, such as the salivary microbiome, have been shown to be different between omnivores and vegan dieters107, opening new avenues for research on adaptable mechanisms related to dietary intake.
A continuum in microbial diversity dependent on diet
Plant-based diets are supposed to be linked to a specific microbial profile, with a vegan profile being most different from an omnivore, but not always different from a vegetarian profile (reviewed in ref. 15). Some specifically vegan gut microbial characteristics have also been found in a small sample of six obese subjects after 1 month following a vegetarian diet, namely less pathobionts, more protective bacterial species improving lipid metabolism and a reduced level of intestinal inflammation108. Investigating long-term dietary patterns a study found a dose-dependent effect for altered gut microbiota in vegetarians and vegans compared to omnivores depending on the quantity of animal products109. The authors showed that gut microbial profiles of plant-based diets feature the same total number but lower counts of Bacteroides, Bifidobacterium, E. coli and Enterobacteriaceae compared to omnivores, with the biggest difference to vegans. Still today it remains unclear, what this shift in bacterial composition means in functional terms, prompting the field to develop more functional analyses.
In a 30-day intervention study, David et al. found that fermentation processes linked to fat and carbohydrate decomposition were related to the abundance of certain microbial species35. They found a strong correlation between fiber intake and Prevotella abundance in the microbial gut. More recently, Prevotella has been associated with plant-based diets110 that are comparable to low-fat/high-fiber diets111 and might be linked to the increased synthesis of short-chain fatty acids (SCFA)112. SCFAs are discussed as putative signaling molecules between the gut microbiome and the receptors, i.e. free fatty acid receptor 2 (FFA2)51, found in host cells across different tissues113 and could therefore be one potential mechanism of microbiome−host communication.
The underlying mechanisms of nutrient decomposition by Prevotella and whether abundant Prevotella populations in the gut are beneficial for overall health remain unknown. Yet it seems possible that an increased fiber intake and therefore higher Prevotella abundance such as associated with plant-based diets is beneficial for regulating glycemic control and keeping inflammatory processes within normal levels, possibly due to reduced appetite and lower energy intake mediated by a higher fiber content114. Moreover, it has been brought forward that the microbiome might influence bodily homeostatic control, suggesting a role for the gut microbiota in whole-body control mechanisms on the systemic level. Novel strategies aim to develop gut-microbiota-based therapies to improve bodily states, e.g. glycemic control115, based on inducing microbial changes and thereby eliciting higher-level changes in homeostasis. While highly speculative, such strategies could in theory also exert changes on the brain level, which will be discussed next in the light of a bi-directional feedback between the gut and the brain.
Effects on cognition and behavior linking diet and cognition via the microbiome−gut−brain axis
While the number of interventional studies focusing on cognitive and mental health outcomes after adopting plant-based diets overall is very limited (see Section I above), one underlying mechanism of how plant-based diets may affect mood could involve signaling pathways on the microbiome−gut−brain axis116,117,118,119. A recent 4-week intervention RCT showed that probiotic administration compared to placebo and no intervention modulated brain activity during emotional decision-making and emotional recognition tasks117. In chronic depression it has been proposed that immunoglobulin A and M antibodies are synthesized by the host in response to gut commensals and are linked to depressive symptoms120. Whether the identified gram-negative bacteria might also play a role in plant-based diets remains to be explored. A meta-analysis on five studies concluded that probiotics may mediate an alleviating effect on depression symptomatic121—however, sample sizes remained rather small (n < 100) and no long-term effects were tested (up to 8 weeks).
Currently, several studies aim to identify microbial profiles in relation to disease and how microbial data can be used on a multimodal way to improve functional resolution, e.g. characterizing microbial profiles of individuals suffering from type-1 diabetes122. Yet, evidence for specific effects of diet on cognitive functions and behavior through changes in the microbiome remains scarce. A recent study indicated the possibility that our food choices determine the quantity and quality of neurotransmitter-precursor levels that we ingest, which in turn might influence behavior, as shown by lower fairness during a money-redistribution task, called the ultimatum game, after a high-carbohydrate/protein ratio breakfast than after a low-ratio breakfast123. Strang et al. found that precursor forms of serotonin and dopamine, measured in blood serum, predicted behavior in this task, and precursor concentrations were dependent on the nutrient profile of the consumed meal before the task. Also on a cross-sectional level tryptophan metabolites from fecal samples have been associated with amygdala-reward network functional connectivity124. On top of the dietary composition per se, the microbiota largely contributes to neurotransmitter precursor concentrations; thus, in addition to measuring neurotransmitter precursors in the serum, metabolomics on fecal samples would be helpful to further understand the functional role of the gut microbiota in neurotransmitter biosynthesis and regulation125.
Indicating the relevance of gut microbiota for cognition, a first human study assessing cognitive tests and brain imaging could distinguish obese from nonobese individuals using a microbial profile126. The authors found a specific microbiotic profile, particularly defined by Actinobacteria phylum abundance, that was associated with microstructural properties in the hypothalamus and in the caudate nucleus. Further, a preclinical study tested whether probiotics could enhance cognitive function in healthy subjects, showing small effects on improved memory performance and reduced stress levels127.
A recent study could show that microbial composition influences cerebral amyloidogenesis in a mouse model for Alzheimer's disease128. Health status of the donor mouse seemingly mattered: fecal transplants from transgenic mice had a larger impact on amyloid beta proliferation in the brain compared to wild-type feces. Translational interpretations to humans should be done with caution if at all—yet the results remain elucidative for showing a link between the gut microbiome and brain metabolism.
The evidence for effects of strictly plant-based diets on cognition is very limited. For other plant-based diets such as the Mediterranean diet or DASH diet, there are more available studies that indicate protective effects on cardiovascular and brain health in the aging population (reviewed in refs. 129,130). Several attempts have been made to clarify potential underlying mechanisms, for example using supplementary plant polyphenols, fish/fish-oil consumption or whole dietary pattern change in RCTs131,132,133,134,135,136,137, yet results are not always equivocal and large-scale intervention studies have yet to be completed.
The overall findings of this paragraph add to the evidence that microbial diversity may be associated with brain health, although underlying mechanisms and candidate signaling molecules remain unknown.
Conclusion
Based on this systematic review of randomized clinical trials, there is an overall robust support for beneficial effects of a plant-based diet on metabolic measures in health and disease. However, the evidence for cognitive and mental effects of a plant-based diet is still inconclusive. Also, it is not clear whether putative effects are due to the diet per se, certain nutrients of the diet (or the avoidance of certain animal-based nutrients) or other factors associated with vegetarian/vegan diets. Evolving concepts argue that emotional distress and mental illnesses are linked to the role of microbiota in neurological function and can be potentially treated via microbial intervention strategies19. Moreover, it has been claimed that certain diseases, such as obesity, are caused by a specific microbial composition138, and that a balanced gut microbiome is related to healthy ageing111. In this light, it seems possible that a plant-based diet is able to influence brain function by still unclear underlying mechanisms of an altered microbial status and systemic metabolic alterations. However, to our knowledge there are no studies linking plant-based diets and cognitive abilities on a neural level, which are urgently needed, due to the hidden potential as a dietary therapeutic tool. Also, further studies are needed to disentangle motivational beliefs on a psychological level that lead to a change in diet from causal effects on the body and the brain mediated e.g., by metabolic alterations or a change in the gut microbiome.
References
- 1.
GOV.UK. National Diet and Nutrition Survey: headline results from years 1, 2 and 3 (combined) of the rolling programme 2008/09–2010/11. https://www.gov.uk/government/statistics/national-diet-and-nutrition-survey-headline-results-from-years-1-2-and-3-combined-of-the-rolling-programme-200809-201011 (2012).
- 2.
V. E. B. U. Deutschland & Joy, S. Anzahl der Veganer und Vegetarier in Deutschland. Stand 31, 2016 (2015).
- 3.
Mensink, G., Barbosa, C. L. & Brettschneider, A.-K. Verbreitung der vegetarischen Ernährungsweise in Deutschland 1, (2016).
- 4.
The Vegetarian Resource Group. How many adults in the U.S. are vegetarian and vegan? http://www.vrg.org/nutshell/Polls/2016_adults_veg.htm (2016).
- 5.
Rosenfeld, D. L. & Burrow, A. L. Vegetarian on purpose: understanding the motivations of plant-based dieters. Appetite 116, 456–463 (2017).
PubMed Article Google Scholar
- 6.
Orlich, M. J. et al. Vegetarian dietary patterns and mortality in Adventist Health Study 2. JAMA Intern. Med. 173, 1230–1238 (2013).
CAS PubMed PubMed Central Article Google Scholar
- 7.
Le, L. T. & Sabaté, J. Beyond meatless, the health effects of vegan diets: findings from the Adventist cohorts. Nutrients 6, 2131–2147 (2014).
PubMed PubMed Central Article Google Scholar
- 8.
Mihrshahi, S. et al. Vegetarian diet and all-cause mortality: evidence from a large population-based Australian cohort-the 45 and up study. Prev. Med. 97, 1–7 (2017).
PubMed Article Google Scholar
- 9.
Key, T. J. et al. Mortality in British vegetarians: results from the European Prospective Investigation into Cancer and Nutrition (EPIC-Oxford). Am. J. Clin. Nutr. 89, 1613S–1619S (2009).
CAS PubMed Article Google Scholar
- 10.
Fung, T. T. et al. Low-carbohydrate diets and all-cause and cause-specific mortalitytwo cohort studies. Ann. Intern. Med. 153, 289–298 (2010).
PubMed PubMed Central Article Google Scholar
- 11.
Song, M. et al. Association of animal and plant protein intake with all-cause and cause-specific mortality. JAMA Intern. Med. 176, 1453–1463 (2016).
PubMed PubMed Central Article Google Scholar
- 12.
Hu, F. B. Plant-based foods and prevention of cardiovascular disease: an overview. Am. J. Clin. Nutr. 78, 544S–551S (2003).
CAS PubMed Article Google Scholar
- 13.
Tonstad, S., Butler, T., Yan, R. & Fraser, G. E. Type of vegetarian diet, body weight, and prevalence of type 2 diabetes. Diabetes Care 32, 791–796 (2009).
PubMed PubMed Central Article Google Scholar
- 14.
McEvoy, C. T., Temple, N. & Woodside, J. V. Vegetarian diets, low-meat diets and health: a review. Public Health Nutr. 15, 2287–2294 (2012).
PubMed Article Google Scholar
- 15.
Glick-Bauer, M. & Yeh, M.-C. The health advantage of a vegan diet: exploring the gut microbiota connection. Nutrients 6, 4822–4838 (2014).
PubMed PubMed Central Article Google Scholar
- 16.
Appleby, P. N. & Key, T. J. The long-term health of vegetarians and vegans. Proc. Nutr. Soc. 75, 287–293 (2016).
PubMed Article Google Scholar
- 17.
Eichelmann, F., Schwingshackl, L., Fedirko, V. & Aleksandrova, K. Effect of plant‐based diets on obesity‐related inflammatory profiles: a systematic review and meta‐analysis of intervention trials. Obes. Rev. 17, 1067–1079 (2016).
CAS PubMed Article Google Scholar
- 18.
McMacken, M. & Shah, S. A plant-based diet for the prevention and treatment of type 2 diabetes. J. Geriatr. Cardiol. 14, 342 (2017).
CAS PubMed PubMed Central Google Scholar
- 19.
Rogers, G. B. et al. From gut dysbiosis to altered brain function and mental illness: mechanisms and pathways. Mol. Psychiatry 21, 738–748 (2016).
CAS PubMed PubMed Central Article Google Scholar
- 20.
Hibbeln, J. R., Northstone, K., Evans, J. & Golding, J. Vegetarian diets and depressive symptoms among men. J. Affect Disord. 225, 13–17 (2018).
PubMed Article Google Scholar
- 21.
Forestell, C. A. & Nezlek, J. B. Vegetarianism, depression, and the five factor model of personality. Ecol. Food Nutr. 57, 246–259 (2018).
PubMed Article Google Scholar
- 22.
Matta, J. et al. Depressive symptoms and vegetarian diets: results from the constances cohort. Nutrients 10, 1695 (2018).
PubMed Central Article PubMed Google Scholar
- 23.
Agarwal, U. et al. A multicenter randomized controlled trial of a nutrition intervention program in a multiethnic adult population in the corporate setting reduces depression and anxiety and improves quality of life: the GEICO study. Am. J. Health Promot. 29, 245–254 (2015).
PubMed Article Google Scholar
- 24.
Beezhold, B., Radnitz, C., Rinne, A. & DiMatteo, J. Vegans report less stress and anxiety than omnivores. Nutr. Neurosci. 18, 289–296 (2015).
PubMed Article Google Scholar
- 25.
Barnard, N. D., Levin, S. M. & Yokoyama, Y. A systematic review and meta-analysis of changes in body weight in clinical trials of vegetarian diets. J. Acad. Nutr. Diet. 115, 954–969 (2015).
PubMed Article Google Scholar
- 26.
Huang, R.-Y., Huang, C.-C., Hu, F. B. & Chavarro, J. E. Vegetarian diets and weight reduction: a meta-analysis of randomized controlled trials. J. Gen. Intern. Med. 31, 109–116 (2016).
PubMed Article Google Scholar
- 27.
Benatar, J. R. & Stewart, R. A. H. Cardiometabolic risk factors in vegans: a meta-analysis of observational studies. PLoS ONE 13, e0209086 (2018).
CAS PubMed PubMed Central Article Google Scholar
- 28.
Lee, Y.-M. et al. Effect of a brown rice based vegan diet and conventional diabetic diet on glycemic control of patients with type 2 diabetes: a 12-week randomized clinical trial. PLoS ONE 11, e0155918 (2016).
PubMed PubMed Central Article CAS Google Scholar
- 29.
Jenkins, D. J. A. et al. Effect of a 6-month vegan low-carbohydrate ('Eco-Atkins') diet on cardiovascular risk factors and body weight in hyperlipidaemic adults: a randomised controlled trial. BMJ Open 4, e003505 (2014).
PubMed PubMed Central Article Google Scholar
- 30.
Jenkins, D. J. A. et al. The effect of a plant-based low-carbohydrate ("Eco-Atkins") diet on body weight and blood lipid concentrations in hyperlipidemic subjects. Arch. Intern. Med. 169, 1046–1054 (2009).
CAS PubMed Article Google Scholar
- 31.
Barnard, N. D. et al. A low-fat vegan diet and a conventional diabetes diet in the treatment of type 2 diabetes: a randomized, controlled, 74-wk clinical trial. Am. J. Clin. Nutr. https://doi.org/10.3945/ajcn.2009.26736H (2009).
CAS PubMed PubMed Central Article Google Scholar
- 32.
Kahleova, H., Hill M. & Pelikánova, T. Vegetarian vs. conventional diabetic diet—a 1-year follow-up. Cor Vasa 56. https://doi.org/10.1016/j.crvasa.2013.12.004 (2016).
Article Google Scholar
- 33.
Turner-McGrievy, G. M., Davidson, C. R., Wingard, E. E., Wilcox, S. & Frongillo, E. A. Comparative effectiveness of plant-based diets for weight loss: a randomized controlled trial of five different diets. Nutrition 31, 350–358 (2015).
PubMed Article Google Scholar
- 34.
Wing, R. R. & Phelan, S. Long-term weight loss maintenance. Am. J. Clin. Nutr. 82, 222S–225S (2005).
CAS PubMed Article PubMed Central Google Scholar
- 35.
David, L. A. et al. Diet rapidly and reproducibly alters the human gut microbiome. Nature 505, 559–563 (2014).
CAS PubMed Article Google Scholar
- 36.
Wu, G. D. et al. Linking long-term dietary patterns with gut microbial enterotypes. Science (80-) 334, 105–108 (2011).
CAS Article Google Scholar
- 37.
Kaartinen, K. et al. Vegan diet alleviates fibromyalgia symptoms. Scand. J. Rheumatol. 29, 308–313 (2000).
CAS PubMed Article Google Scholar
- 38.
Yadav, V. et al. Low-fat, plant-based diet in multiple sclerosis: a randomized controlled trial. Mult. Scler. Relat. Disord. 9, 80–90 (2016).
PubMed Article PubMed Central Google Scholar
- 39.
Rauma, A. L., Nenonen, M., Helve, T. & Hänninen, O. Effect of a strict vegan diet on energy and nutrient intakes by Finnish rheumatoid patients. Eur. J. Clin. Nutr. 47, 747–749 (1993).
CAS PubMed Google Scholar
- 40.
Elkan, A.-C. et al. Gluten-free vegan diet induces decreased LDL and oxidized LDL levels and raised atheroprotective natural antibodies against phosphorylcholine in patients with rheumatoid arthritis: a randomized study. Arthritis Res. Ther. 10, R34 (2008).
PubMed PubMed Central Article CAS Google Scholar
- 41.
Karlsson, J. et al. Predictors and effects of long-term dieting on mental well-being and weight loss in obese women. Appetite 23, 15–26 (1994).
CAS PubMed Article PubMed Central Google Scholar
- 42.
Beezhold, B. L. & Johnston, C. S. Restriction of meat, fish, and poultry in omnivores improves mood: a pilot randomized controlled trial. Nutr. J. 11, 9 (2012).
CAS PubMed PubMed Central Article Google Scholar
- 43.
Yokoyama, Y., Barnard, N. D., Levin, S. M. & Watanabe, M. Vegetarian diets and glycemic control in diabetes: a systematic review and meta-analysis. Cardiovasc. Diagn. Ther. 4, 373–382 (2014).
PubMed PubMed Central Google Scholar
- 44.
Sutliffe, J. T., Wilson, L. D., de Heer, H. D., Foster, R. L. & Carnot, M. J. C-reactive protein response to a vegan lifestyle intervention. Complement Ther. Med. 23, 32–37 (2015).
PubMed Article PubMed Central Google Scholar
- 45.
Strasser, B., Gostner, J. M. & Fuchs, D. Mood, food, and cognition: role of tryptophan and serotonin. Curr. Opin. Clin. Nutr. Metab. Care 19, 55–61 (2016).
CAS PubMed Article PubMed Central Google Scholar
- 46.
O'Mahony, S. M., Clarke, G., Borre, Y. E., Dinan, T. G. & Cryan, J. F. Serotonin, tryptophan metabolism and the brain-gut-microbiome axis. Behav. Brain Res. 277, 32–48 (2015).
PubMed Article CAS PubMed Central Google Scholar
- 47.
Davey, G. K. et al. EPIC–Oxford: lifestyle characteristics and nutrient intakes in a cohort of 33 883 meat-eaters and 31 546 non meat-eaters in the UK. Public Health Nutr. 6, 259–268 (2003).
PubMed Article PubMed Central Google Scholar
- 48.
Schüpbach, R., Wegmüller, R., Berguerand, C., Bui, M. & Herter-Aeberli, I. Micronutrient status and intake in omnivores, vegetarians and vegans in Switzerland. Eur. J. Nutr. 56, 283–293 (2017).
PubMed Article CAS PubMed Central Google Scholar
- 49.
Clarys, P. et al. Comparison of nutritional quality of the vegan, vegetarian, semi-vegetarian, pesco-vegetarian and omnivorous diet. Nutrients 6, 1318–1332 (2014).
PubMed PubMed Central Article CAS Google Scholar
- 50.
Park, J. E., Miller, M., Rhyne, J., Wang, Z. & Hazen, S. L. Differential effect of short-term popular diets on TMAO and other cardio-metabolic risk markers. Nutr. Metab. Cardiovasc. Dis. 29, 513–517 (2019).
CAS PubMed Article PubMed Central Google Scholar
- 51.
Psichas, A. et al. The short chain fatty acid propionate stimulates GLP-1 and PYY secretion via free fatty acid receptor 2 in rodents. Int J. Obes. 39, 424 (2015).
CAS Article Google Scholar
- 52.
Lin, H. V. et al. Butyrate and propionate protect against diet-induced obesity and regulate gut hormones via free fatty acid receptor 3-independent mechanisms. PLoS ONE 7, e35240 (2012).
CAS PubMed PubMed Central Article Google Scholar
- 53.
Canfora, E. E., Jocken, J. W. & Blaak, E. E. Short-chain fatty acids in control of body weight and insulin sensitivity. Nat. Rev. Endocrinol. 11, 577 (2015).
CAS PubMed Article PubMed Central Google Scholar
- 54.
Guo, Y. et al. Physiological evidence for the involvement of peptide YY in the regulation of energy homeostasis in humans. Obesity 14, 1562–1570 (2006).
CAS PubMed Article PubMed Central Google Scholar
- 55.
Holzer, P., Reichmann, F. & Farzi, A. Neuropeptide Y, peptide YY and pancreatic polypeptide in the gut–brain axis. Neuropeptides 46, 261–274 (2012).
CAS PubMed PubMed Central Article Google Scholar
- 56.
Kendall, C. W. C., Esfahani, A. & Jenkins, D. J. A. The link between dietary fibre and human health. Food Hydrocoll. 24, 42–48 (2010).
CAS Article Google Scholar
- 57.
Reynolds, A. et al. Carbohydrate quality and human health: a series of systematic reviews and meta-analyses. Lancet. https://doi.org/10.1016/S0140-6736(18)31809-9 (2019).
CAS Article Google Scholar
- 58.
Menni, C. et al. Gut microbiome diversity and high-fibre intake are related to lower long-term weight gain. Int. J. Obes. 41, 1099 (2017).
CAS Article Google Scholar
- 59.
Van Gaal, L. F., Mertens, I. L. & Christophe, E. Mechanisms linking obesity with cardiovascular disease. Nature 444, 875 (2006).
PubMed Article CAS PubMed Central Google Scholar
- 60.
Ferreira, C. M. et al. The central role of the gut microbiota in chronic inflammatory diseases. J. Immunol. Res. 2014, https://doi.org/10.1155/2014/689492 (2014).
Article CAS Google Scholar
- 61.
Wersching, H. et al. Serum C-reactive protein is linked to cerebral microstructural integrity and cognitive function. Neurology 74, 1022–1029 (2010).
CAS PubMed Article PubMed Central Google Scholar
- 62.
Gu, Y. et al. Circulating inflammatory biomarkers in relation to brain structural measurements in a non-demented elderly population. Brain Behav. Immun. 65, 150–160 (2017).
CAS PubMed PubMed Central Article Google Scholar
- 63.
Lampe, L. et al. Visceral obesity relates to deep white matter hyperintensities via inflammation. Ann. Neurol. 85, 194–203 (2018).
Google Scholar
- 64.
Schmidt, R. et al. Early inflammation and dementia: a 25‐year follow‐up of the Honolulu‐Asia Aging Study. Ann. Neurol. 52, 168–174 (2002).
PubMed Article PubMed Central Google Scholar
- 65.
Rosano, C., Marsland, A. L. & Gianaros, P. J. Maintaining brain health by monitoring inflammatory processes: a mechanism to promote successful aging. Aging Dis. 3, 16 (2012).
PubMed PubMed Central Google Scholar
- 66.
Tangney, C. C. et al. Relation of DASH-and Mediterranean-like dietary patterns to cognitive decline in older persons. Neurology 83, 1410–1416 (2014).
CAS PubMed PubMed Central Article Google Scholar
- 67.
Craddock, J. C., Probst, Y. & Peoples, G. Vegetarian nutrition—comparing physical performance of omnivorous and vegetarian athletes. J. Nutr. Intermed. Metab. 4, 19 (2016).
Article Google Scholar
- 68.
Liu, R. H. Health benefits of fruit and vegetables are from additive and synergistic combinations of phytochemicals. Am. J. Clin. Nutr. 78, 517S–520S (2003).
CAS PubMed Article PubMed Central Google Scholar
- 69.
Boffetta, P. et al. Fruit and vegetable intake and overall cancer risk in the European Prospective Investigation into Cancer and Nutrition (EPIC). J. Natl. Cancer Inst. 102, 529–537 (2010).
CAS PubMed Article PubMed Central Google Scholar
- 70.
Reczek, C. R. & Chandel, N. S. Revisiting vitamin C and cancer. Science (80-) 350, 1317–1318 (2015).
Article Google Scholar
- 71.
Probst, Y. C., Guan, V. X. & Kent, K. Dietary phytochemical intake from foods and health outcomes: a systematic review protocol and preliminary scoping. BMJ Open 7, e013337 (2017).
PubMed PubMed Central Article Google Scholar
- 72.
Hartmann, R. & Meisel, H. Food-derived peptides with biological activity: from research to food applications. Curr. Opin. Biotechnol. 18, 163–169 (2007).
CAS PubMed Article PubMed Central Google Scholar
- 73.
Tillisch, K. et al. Consumption of fermented milk product with probiotic modulates brain activity. Gastroenterology 144, 1394–1401 (2013).
CAS PubMed Article PubMed Central Google Scholar
- 74.
Gibson, G. R. et al. Expert consensus document: The International Scientific Association for Probiotics and Prebiotics (ISAPP) consensus statement on thedefinition and scope of prebiotics. Nat. Rev. Gastroenterol. Hepatol. 14, 491 (2017).
PubMed Article PubMed Central Google Scholar
- 75.
Nisha, A. R. Antibiotic residues-a global health hazard. Vet. World 1, 375–377 (2008).
Article Google Scholar
- 76.
Wang, H. et al. Antibiotic residues in meat, milk and aquatic products in Shanghai and human exposure assessment. Food Control 80, 217–225 (2017).
CAS Article Google Scholar
- 77.
Bertazzi, P. A. et al. Health effects of dioxin exposure: a 20-year mortality study. Am. J. Epidemiol. 153, 1031–1044 (2001).
CAS PubMed Article PubMed Central Google Scholar
- 78.
Bouvard, V. et al. Carcinogenicity of consumption of red and processed meat. Lancet Oncol. 16, 1599–1600 (2015).
PubMed Article PubMed Central Google Scholar
- 79.
Van Audenhaege, M. et al. Impact of food consumption habits on the pesticide dietary intake: comparison between a French vegetarian and the general population. Food Addit. Contam. 26, 1372–1388 (2009).
Article CAS Google Scholar
- 80.
Jacobs, D. R. & Tapsell, L. C. Food synergy: the key to a healthy diet. Proc. Nutr. Soc. 72, 200–206 (2013).
PubMed Article Google Scholar
- 81.
Kawano, Y. & Yanai, K. Foodcam: a real-time food recognition system on a smartphone. Multimed. Tools Appl. 74, 5263–5287 (2015).
Article Google Scholar
- 82.
Garcia-Perez, I. et al. Objective assessment of dietary patterns by use of metabolic phenotyping: a randomised, controlled, crossover trial. Lancet Diabetes Endocrinol. 5, 184–195 (2017).
PubMed PubMed Central Article Google Scholar
- 83.
Turner-McGrievy, G. M. et al. Changes in nutrient intake and dietary quality among participants with type 2 diabetes following a low-fat vegan diet or a conventional diabetes diet for 22 weeks. J. Am. Diet. Assoc. 108, 1636–1645 (2008).
PubMed Article Google Scholar
- 84.
Gilsing, A. M. J. et al. Serum concentrations of vitamin B12 and folate in British male omnivores, vegetarians and vegans: results from a cross-sectional analysis of the EPIC-Oxford cohort study. Eur. J. Clin. Nutr. 64, 933–939 (2010).
CAS PubMed PubMed Central Article Google Scholar
- 85.
Allen, L. H. How common is vitamin B-12 deficiency? Am. J. Clin. Nutr. 89, 693S–696S (2008).
PubMed Article CAS Google Scholar
- 86.
Pawlak, R., Parrott, S. J., Raj, S., Cullum-Dugan, D. & Lucus, D. How prevalent is vitamin B12 deficiency among vegetarians? Nutr. Rev. 71, 110–117 (2013).
PubMed Article Google Scholar
- 87.
Rizzo, G. et al. Vitamin B12 among vegetarians: status, assessment and supplementation. Nutrients 8, 767 (2016).
PubMed Central Article CAS PubMed Google Scholar
- 88.
Stabler, S. P. Vitamin B12 deficiency. N. Engl. J. Med. 368, 149–160 (2013).
CAS PubMed Article Google Scholar
- 89.
Köbe, T. et al. Vitamin B-12 concentration, memory performance, and hippocampal structure in patients with mild cognitive impairment, 2. Am. J. Clin. Nutr. 103, 1045–1054 (2016).
PubMed Article CAS Google Scholar
- 90.
Ganguly, P. & Alam, S. F. Role of homocysteine in the development of cardiovascular disease. Nutr. J. 14, 6 (2015).
PubMed PubMed Central Article CAS Google Scholar
- 91.
McCaddon, A., Regland, B., Hudson, P. & Davies, G. Functional vitamin B12 deficiency and Alzheimer disease. Neurology 58, 1395–1399 (2002).
CAS PubMed Article Google Scholar
- 92.
Moore, E. et al. Cognitive impairment and vitamin B12: a review. Int. Psychogeriatr. 24, 541–556 (2012).
PubMed Article Google Scholar
- 93.
Spence, J. D. Metabolic vitamin B12 deficiency: a missed opportunity to prevent dementia and stroke. Nutr. Res. 36, 109–116 (2016).
CAS PubMed Article Google Scholar
- 94.
Nexo, E. & Hoffmann-Lücke, E. Holotranscobalamin, a marker of vitamin B-12 status: analytical aspects and clinical utility. Am. J. Clin. Nutr. 94, 359S–365S (2011).
CAS PubMed PubMed Central Article Google Scholar
- 95.
Haider, L. M., Schwingshackl, L., Hoffmann, G. & Ekmekcioglu, C. The effect of vegetarian diets on iron status in adults: a systematic review and meta-analysis. Crit. Rev. Food Sci. Nutr. 58, 1359–1374 (2018).
CAS PubMed Article Google Scholar
- 96.
Lozoff, B. & Georgieff, M. K. et al. Iron deficiency and brain development. Semin. Pediatr. Neurol. 13, 158–165 (2006).
PubMed Article Google Scholar
- 97.
Ayton, S. et al. Brain iron is associated with accelerated cognitive decline in people with Alzheimer pathology. Mol. Psychiatry 1, https://doi.org/10.1038/s41380-019-0375-7 (2019).
- 98.
Murray-Kolb, L. E. & Beard, J. L. Iron treatment normalizes cognitive functioning in young women. Am. J. Clin. Nutr. 85, 778–787 (2007).
CAS PubMed Article Google Scholar
- 99.
Beard, J. Iron deficiency alters brain development and functioning. J. Nutr. 133, 1468S–1472S (2003).
CAS PubMed Article Google Scholar
- 100.
Melina, V., Craig, W. & Levin, S. Position of the Academy of Nutrition and Dietetics: vegetarian diets. J. Acad. Nutr. Diet. 116, 1970–1980 (2016).
PubMed Article Google Scholar
- 101.
Richter, M. et al. For the German Nutrition Society (DGE)(2016) Vegan diet. Position of the German Nutrition Society (DGE). Ernaehrungsumschau 63, 92–102 (2016).
Google Scholar
- 102.
Peterson, J. et al. The NIH human microbiome project. Genome Res. 19, 2317–2323 (2009).
PubMed PubMed Central Article CAS Google Scholar
- 103.
Arumugam, M. et al. Enterotypes of the human gut microbiome. Nature 473, 174–180 (2013).
Article CAS Google Scholar
- 104.
Bamberger, C. et al. A walnut-enriched diet affects gut microbiome in healthy Caucasian subjects: a randomized, controlled trial. Nutrients 10, 244 (2018).
PubMed Central Article CAS PubMed Google Scholar
- 105.
Holscher, H. D. et al. Walnut consumption alters the gastrointestinal microbiota, microbially derived secondary bile acids, and health markers in healthy adults: a randomized controlled trial. J. Nutr. 148, 861–867 (2018).
PubMed PubMed Central Article Google Scholar
- 106.
Hjorth, M. F. et al. Pre-treatment microbial Prevotella-to-Bacteroides ratio, determines body fat loss success during a 6-month randomized controlled diet intervention. Int J. Obes. 42, 580 (2018).
CAS Article Google Scholar
- 107.
Hansen, T. H. et al. Impact of a vegan diet on the human salivary microbiota. Sci. Rep. 8, 5847 (2018).
PubMed PubMed Central Article CAS Google Scholar
- 108.
Kim, M., Hwang, S., Park, E. & Bae, J. Strict vegetarian diet improves the risk factors associated with metabolic diseases by modulating gut microbiota and reducing intestinal inflammation. Environ. Microbiol. Rep. 5, 765–775 (2013).
CAS PubMed Article Google Scholar
- 109.
Zimmer, J. et al. A vegan or vegetarian diet substantially alters the human colonic faecal microbiota. Eur. J. Clin. Nutr. 66, 53–60 (2012).
CAS PubMed Article Google Scholar
- 110.
De Filippis, F., Pellegrini, N., Laghi, L., Gobbetti, M. & Ercolini, D. Unusual sub-genus associations of faecal Prevotella and Bacteroides with specific dietary patterns. Microbiome 4, 57 (2016).
PubMed PubMed Central Article Google Scholar
- 111.
Kumar, M., Babaei, P., Ji, B. & Nielsen, J. Human gut microbiota and healthy aging: Recent developments and future prospective. Nutr. Health Aging 4, 3–16 (2016).
Article Google Scholar
- 112.
Wu, G. D. et al. Comparative metabolomics in vegans and omnivores reveal constraints on diet-dependent gut microbiota metabolite production. Gut 65, 63–72 (2014).
PubMed PubMed Central Article CAS Google Scholar
- 113.
Morrison, D. J. & Preston, T. Formation of short chain fatty acids by the gut microbiota and their impact on human metabolism. Gut Microbes 7, 189–200 (2016).
PubMed PubMed Central Article Google Scholar
- 114.
Wanders, A. J. et al. Effects of dietary fibre on subjective appetite, energy intake and body weight: a systematic review of randomized controlled trials. Obes. Rev. 12, 724–739 (2011).
CAS PubMed PubMed Central Google Scholar
- 115.
Brunkwall, L. & Orho-Melander, M. The gut microbiome as a target for prevention and treatment of hyperglycaemia in type 2 diabetes: from current human evidence to future possibilities. Diabetologia 60, 943–951 (2017).
CAS PubMed PubMed Central Article Google Scholar
- 116.
Lach, G., Schellekens, H., Dinan, T. G. & Cryan, J. F. Anxiety, depression, and the microbiome: a role for gut peptides. Neurotherapeutics 15, 36–59 (2018).
CAS PubMed Article PubMed Central Google Scholar
- 117.
Bagga, D. et al. Influence of 4-week multi-strain probiotic administration on resting-state functional connectivity in healthy volunteers. Eur. J. Nutr. 58, 1821–1827 (2018).
PubMed PubMed Central Article CAS Google Scholar
- 118.
Foster, J. A. & Neufeld, K.-A. M. Gut–brain axis: how the microbiome influences anxiety and depression. Trends Neurosci. 36, 305–312 (2013).
CAS PubMed PubMed Central Article Google Scholar
- 119.
Saulnier, D. M. et al. The intestinal microbiome, probiotics and prebiotics in neurogastroenterology. Gut Microbes 4, 17–27 (2013).
PubMed PubMed Central Article Google Scholar
- 120.
Maes, M., Kubera, M., Leunis, J.-C. & Berk, M. Increased IgA and IgM responses against gut commensals in chronic depression: further evidence for increased bacterial translocation or leaky gut. J. Affect Disord. 141, 55–62 (2012).
CAS PubMed Article Google Scholar
- 121.
Huang, R., Wang, K. & Hu, J. Effect of probiotics on depression: a systematic review and meta-analysis of randomized controlled trials. Nutrients 8, 483 (2016).
PubMed Central Article PubMed Google Scholar
- 122.
Heintz-Buschart, A. et al. Integrated multi-omics of the human gut microbiome in a case study of familial type 1 diabetes. Nat. Microbiol. 2, 16180 (2016).
CAS PubMed Article Google Scholar
- 123.
Strang, S. et al. Impact of nutrition on social decision making. Proc. Natl Acad. Sci. 114, 6510–6514 (2017).
CAS PubMed Article Google Scholar
- 124.
Osadchiy, V. et al. Correlation of tryptophan metabolites with connectivity of extended central reward network in healthy subjects. PLoS ONE 13, e0201772 (2018).
PubMed PubMed Central Article CAS Google Scholar
- 125.
Franzosa, E. A. et al. Sequencing and beyond: integrating molecular'omics' for microbial community profiling. Nat. Rev. Microbiol. 13, 360–372 (2015).
CAS PubMed PubMed Central Article Google Scholar
- 126.
Fernandez-Real, J.-M. et al. Gut microbiota interacts with brain microstructure and function. J. Clin. Endocrinol. Metab. 100, 4505–4513 (2015).
CAS PubMed Article Google Scholar
- 127.
Allen, A. P. et al. Bifidobacterium longum 1714 as a translational psychobiotic: modulation of stress, electrophysiology and neurocognition in healthy volunteers. Transl. Psychiatry 6, e939 (2016).
CAS PubMed PubMed Central Article Google Scholar
- 128.
Harach, T. et al. Reduction of Abeta amyloid pathology in APPPS1 transgenic mice in the absence of gut microbiota. Sci. Rep. 7, 41802 (2017).
CAS PubMed PubMed Central Article Google Scholar
- 129.
Huhn, S., Masouleh, S. K., Stumvoll, M., Villringer, A. & Witte, A. V. Components of a Mediterranean diet and their impact on cognitive functions in aging. Front Aging Neurosci 7, 132 (2015).
PubMed PubMed Central Article CAS Google Scholar
- 130.
Larsson, S. C., Wallin, A. & Wolk, A. Dietary approaches to stop hypertension diet and incidence of stroke: results from 2 prospective cohorts. Stroke 47, 986–990 (2016).
CAS PubMed Article Google Scholar
- 131.
van de Rest, O. et al. Effect of fish oil on cognitive performance in older subjects: a randomized, controlled trial. Neurology 71, 430–438 (2008).
PubMed Article CAS PubMed Central Google Scholar
- 132.
Witte, A. V. et al. Long-chain omega-3 fatty acids improve brain function and structure in older adults. Cereb. Cortex 24, 3059–3068 (2013).
PubMed Article PubMed Central Google Scholar
- 133.
Witte, A. V., Kerti, L., Margulies, D. S. & Flöel, A. Effects of resveratrol on memory performance, hippocampal functional connectivity, and glucose metabolism in healthy older adults. J. Neurosci. 34, 7862–7870 (2014).
CAS PubMed PubMed Central Article Google Scholar
- 134.
Brickman, A. M. et al. Enhancing dentate gyrus function with dietary flavanols improves cognition in older adults. Nat. Neurosci. 17, 1798 (2014).
CAS PubMed PubMed Central Article Google Scholar
- 135.
Martínez-González, M. A. et al. Benefits of the Mediterranean diet: insights from the PREDIMED study. Prog. Cardiovasc. Dis. 58, 50–60 (2015).
PubMed Article PubMed Central Google Scholar
- 136.
Huhn, S. et al. Effects of resveratrol on memory performance, hippocampus connectivity and microstructure in older adults—a randomized controlled trial. Neuroimage (2018).
- 137.
Rosenberg, A. et al. Multidomain lifestyle intervention benefits a large elderly population at risk for cognitive decline and dementia regardless of baseline characteristics: The FINGER trial. Alzheimer's. Dement. 14, 263–270 (2018).
Article Google Scholar
- 138.
Turnbaugh, P. J. Microbes and diet-induced obesity: fast, cheap, and out of control. Cell Host Microbe 21, 278–281 (2017).
CAS PubMed PubMed Central Article Google Scholar
- 139.
Turner-Mc Grievy, G. M., Barnard, N. D. & Scialli, A. R. A two-year randomized weight loss trial comparing a vegan diet to a more moderate low-fat diet*. Obesity 15, 2276–2281 (2007).
Article Google Scholar
- 140.
Burke, L. E. et al. A randomized clinical trial of a standard versus vegetarian diet for weight loss: the impact of treatment preference. Int. J. Obes. 32, 166–176 (2008).
CAS Article Google Scholar
- 141.
Barnard, N. D. et al. A low-fat vegan diet and a conventional diabetes diet in the treatment of type 2 diabetes: a randomized, controlled, 74-wk clinical trial. Am. J. Clin. Nutr. 89, 1588S–1596S (2009).
CAS PubMed PubMed Central Article Google Scholar
- 142.
Marniemi, J., Seppänen, A. & Hakala, P. Long-term effects on lipid metabolism of weight reduction on lactovegetarian and mixed diet. Int. J. Obes. 14, 113–125 (1990).
CAS PubMed PubMed Central Google Scholar
- 143.
Acharya, S. D., Brooks, M. M., Evans, R. W., Linkov, F. & Burke, L. E. Weight loss is more important than the diet type in improving adiponectin levels among overweight/obese adults. J. Am. Coll. Nutr. 32, 264–271 (2013).
CAS PubMed Article PubMed Central Google Scholar
- 144.
Wright, N., Wilson, L., Smith, M., Duncan, B. & McHugh, P. The BROAD study: A randomised controlled trial using a whole food plant-based diet in the community for obesity, ischaemic heart disease or diabetes. Nutr. Diabetes 7, e256 (2017).
CAS PubMed PubMed Central Article Google Scholar
- 145.
Turner-McGrievy, G. M., Davidson, C. R., Wingard, E. E. & Billings, D. L. Low glycemic index vegan or low-calorie weight loss diets for women with polycystic ovary syndrome: a randomized controlled feasibility study. Nutr. Res. 34, 552–558 (2014).
CAS PubMed Article PubMed Central Google Scholar
- 146.
Kahleova, H. et al. Vegetarian diet improves insulin resistance and oxidative stress markers more than conventional diet in subjects with Type 2 diabetes. Diabet. Med 28, 549–559 (2011).
CAS PubMed PubMed Central Article Google Scholar
- 147.
Ferdowsian, H. R. et al. A multicomponent intervention reduces body weight and cardiovascular risk at a GEICO corporate site. Am. J. Heal. Promot 24, 384–387 (2010).
Article Google Scholar
- 148.
Mishra, S. et al. A multicenter randomized controlled trial of a plant-based nutrition program to reduce body weight and cardiovascular risk in the corporate setting: the GEICO study. Eur. J. Clin. Nutr. 67, 718 (2013).
CAS PubMed PubMed Central Article Google Scholar
- 149.
Agarwal, U. et al. A multicenter randomized controlled trial of a nutrition intervention program in a multiethnic adult population in the corporate setting reduces depression and anxiety and improves quality of life: the GEICO study. Am. J. Heal. Promot 29, 245–254 (2015).
Article Google Scholar
- 150.
Kahleova, H., Dort, S., Holubkov, R. & Barnard, N. A plant-based high-carbohydrate, low-fat diet in overweight individuals in a 16-week randomized clinical trial: the role of carbohydrates. Nutrients 10, 1302 (2018).
PubMed Central Article CAS Google Scholar
- 151.
Barnard, N., Scialli, A. R., Bertron, P., Hurlock, D. & Edmonds, K. Acceptability of a therapeutic low-fat, vegan diet in premenopausal women. J. Nutr. Educ. 32, 314–319 (2000).
Article Google Scholar
- 152.
Gardner, C. D. et al. The effect of a plant-based diet on plasma lipids in hypercholesterolemic adults: a randomized trial. Ann. Intern. Med. 142, 733 (2005).
Google Scholar
- 153.
Macknin, M. et al. Plant-based, no-added-fat or American Heart Association diets: impact on cardiovascular risk in obese children with hypercholesterolemia and their parents. J. Pediatr. 166, 953–959 (2015).
PubMed PubMed Central Article Google Scholar
- 154.
Sciarrone, S. E. et al. Biochemical and neurohormonal responses to the introduction of a lacto-ovovegetarian diet. J. Hypertens. 11, 849–860 (1993).
CAS PubMed Article PubMed Central Google Scholar
- 155.
Alleman, R. J., Harvey, I. C., Farney, T. M. & Bloomer, R. J. Both a traditional and modified Daniel Fast improve the cardio-metabolic profile in men and women. Lipids Health Dis. 12, 114 (2013).
CAS PubMed PubMed Central Article Google Scholar
- 156.
Neacsu, M., Fyfe, C., Horgan, G. & Johnstone, A. M. Appetite control and biomarkers of satiety with vegetarian (soy) and meat-based high-protein diets for weight loss in obese men: a randomized crossover trial–. Am. J. Clin. Nutr. 100, 548–558 (2014).
CAS PubMed Article PubMed Central Google Scholar
- 157.
Koebnick, C. et al. Double-blind, randomized feedback control fails to improve the hypocholesterolemic effect of a plant-based low-fat diet in patients with moderately elevated total cholesterol levels. Eur. J. Clin. Nutr. 58, 1402 (2004).
CAS PubMed Article Google Scholar
- 158.
Kjeldsen-Kragh, J., Haugen, M., Førre, Ø., Laache, H. & Malt, U. F. Vegetarian diet for patients with rheumatoid arthritis: can the clinical effects be explained by the psychological characteristics of the patients? Rheumatology 33, 569–575 (1994).
CAS Article Google Scholar
- 159.
Bunner, A. E., Agarwal, U., Gonzales, J. F., Valente, F. & Barnard, N. D. Nutrition intervention for migraine: a randomized crossover trial. J. Headache Pain. 15, 69 (2014).
PubMed PubMed Central Article CAS Google Scholar
- 160.
Kahleova, H., Hrachovinova, T., Hill, M. & Pelikanova, T. Vegetarian diet in type 2 diabetes–improvement in quality of life, mood and eating behaviour. Diabet. Med 30, 127–129 (2013).
CAS PubMed Article PubMed Central Google Scholar
- 161.
Turner-McGrievy, G. M. et al. Randomization to plant-based dietary approaches leads to larger short-term improvements in Dietary Inflammatory Index scores and macronutrient intake compared with diets that contain meat. Nutr. Res. 35, 97–106 (2015).
CAS PubMed Article PubMed Central Google Scholar
Download references
Acknowledgements
This work was supported by a scholarship (E.M.) by the German Federal Environmental Foundation and by the grants of the German Research Foundation contract grant number CRC 1052 "Obesity mechanisms" Project A1 (AV) and WI 3342/3-1 (A.V.W.).
Ethics declarations
Conflict of interest
The authors declare that they have no conflict of interest.
Additional information
Publisher's note Springer Nature remains neutral with regard to jurisdictional claims in published maps and institutional affiliations.
Supplementary information
Rights and permissions
Open Access This article is licensed under a Creative Commons Attribution 4.0 International License, which permits use, sharing, adaptation, distribution and reproduction in any medium or format, as long as you give appropriate credit to the original author(s) and the source, provide a link to the Creative Commons license, and indicate if changes were made. The images or other third party material in this article are included in the article's Creative Commons license, unless indicated otherwise in a credit line to the material. If material is not included in the article's Creative Commons license and your intended use is not permitted by statutory regulation or exceeds the permitted use, you will need to obtain permission directly from the copyright holder. To view a copy of this license, visit http://creativecommons.org/licenses/by/4.0/.
Reprints and Permissions
About this article
Cite this article
Medawar, E., Huhn, S., Villringer, A. et al. The effects of plant-based diets on the body and the brain: a systematic review. Transl Psychiatry 9, 226 (2019). https://doi.org/10.1038/s41398-019-0552-0
Download citation
-
Received:
-
Revised:
-
Accepted:
-
Published:
-
DOI : https://doi.org/10.1038/s41398-019-0552-0
Further reading
Whole Food Plant Based Diet Criticism
Source: https://www.nature.com/articles/s41398-019-0552-0#:~:text=However%2C%20a%20major%20criticism%20of,seems%20detrimental%20for%20neurological%20and